The third prize in the DDRN Essay Competition for University Students 2019 was awarded to Andrea Palessandro, Physics, University of Southern Denmark. The Assessment Committee wrote: “Very well written and structured to make an exciting essay, which potentially will address a wide audience…The topic is not for everyday consumption, rather it calls for extended contemplation during a Sunday afternoon.”
“Quantum mechanics is magic” Daniel Greenberger
Prologue: The Conversation
E: … so the electron is a wave, until you measure its position. Then it suddenly becomes a localized particle. That is crazy!
B: I wouldn’t say it like that. I would say that the electron is described by a wavefunction. The wavefunction represents the probability of finding the electron somewhere in space. When you perform a measurement, the electron will appear in some position according to the probability distribution encoded by the wavefunction. See, the wavefunction is not real, it is simply a tool used by us humans to formalize our knowledge about the system.
E: But what is the electron doing when you’re not looking?
B: How should I know? Whatever it pleases. The point is that our knowledge of the system is incomplete, and the more incomplete it is, the more the wavefunction spreads all over space. Only the measurement can clear all doubts about the electron’s whereabouts.
E: But surely, the electron is somewhere even when you are not looking!
B: That is your preconception.
E: Komm schon! Do you really believe that the moon exists only when you look at it?
B: That, my dear friend, is a metaphysical question. We are physicists, we should only concern ourselves with what we can measure.
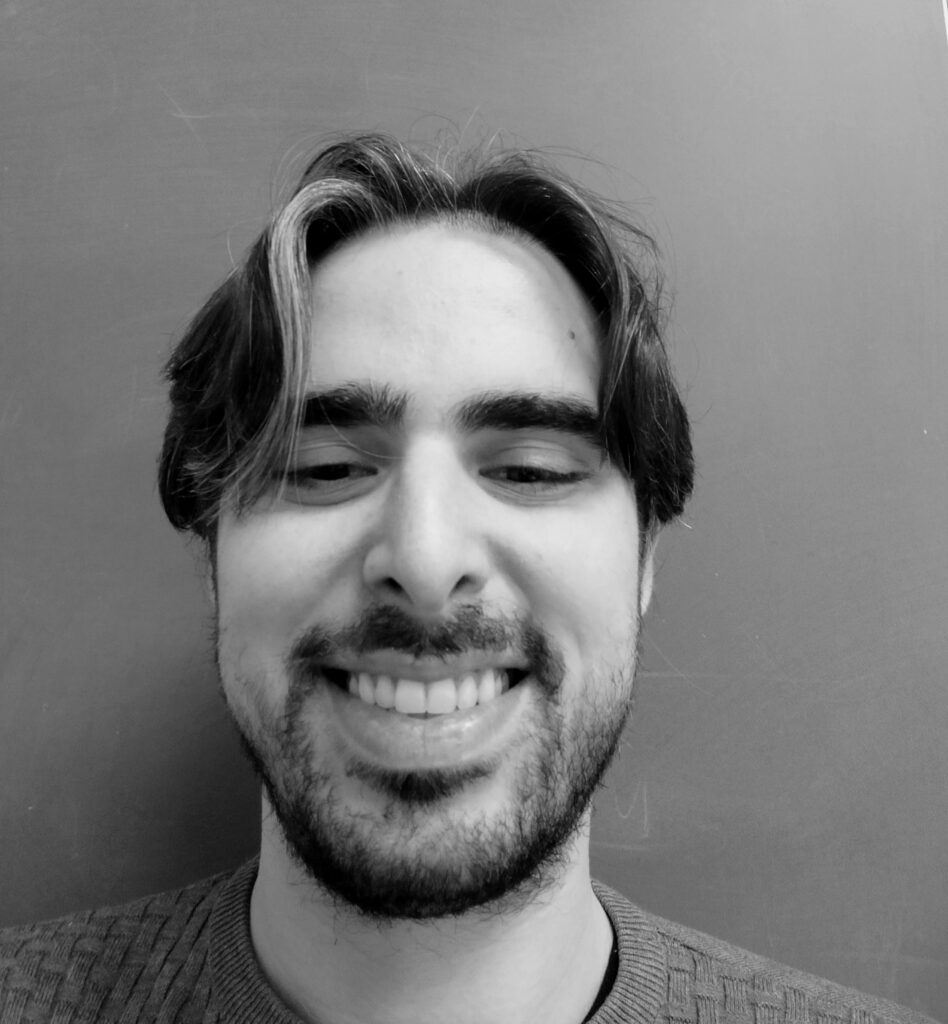
E: And what is a measurement, exactly? When nobody is looking, the wavefunction evolves like a classical wave in a purely deterministic fashion. Yet, it abruptly and randomly collapses when a measurement is performed. Two different evolution laws are needed for the same physical system, depending on whether someone is looking or not. But what is so special about a measurement? Are you perhaps saying that our consciousness somehow affects microscopic phenomena? Or that there is something unique about our experimental instruments that interferes so dramatically on the quantum state of matter? Surely, physicists and their apparatus must be governed by the same quantum mechanical rules that govern everything else in the universe. You must agree on that!
B: I do not. See, my friend, I think that you are way too ambitious. You want to understand the mind of God, but that is just not possible. Not for a physicist at least, and not with the tools of Science. There is a difference between a problem and a mystery. The way quantum mechanics works is a problem that we have just solved. As to what it all means… that is a mystery. It lies beyond the boundary of our limited mental capabilities. We are biological systems, after all. Or do you think that we are angels?
E: Not anymore.
B: Præcis.
The Odd One Out
Since the early days of quantum mechanics, when a similar conversation presumably played out between two giants of physics, our understanding of it has evolved. Quantum mechanics now reign supreme over the world of physics and it is the fundamental ingredient of our most successful theory to date: the Standard Model of particle physics. The Standard Model, together with Einstein’s theory of gravity, General Relativity, allows us to trace back the history of our universe to the very first moments after the Big Bang, the cosmic explosion that set all things in motion.
Despite its obvious successes, quantum mechanics has its flaws, some hidden, some glaring. A chief example is gravity, that has so far resisted an embedding into a quantum structure. The quantum theory of gravity is the current holy grail of theoretical physics, but coming up with a coherent framework for it has proved to be a formidable problem, one that generations of brilliant minds have been unable to crack, although few of them would dispute that a quantum theory of gravitation does exist.
Einstein taught us that gravity is not a force after all, just the manifestation of the curvature of spacetime. It is often said, especially in movies and tv-shows, that spacetime is a continuum. This is true in most cases: spacetime in the solar system is weakly curved (even the Sun is not massive enough to cause a considerable curvature around it) and treating it as continuous is a very good approximation. However, most physicist believe that quantum mechanics, not spacetime, is the most fundamental feature of our universe and therefore even gravity has to exhibit some kind of granularity or, as the jargon goes, quantization. Indeed, the quantization of gravity, its discreteness, is described in terms of gravitons, hypothetical elementary particles that mediate the attractive force and make up the gravitational field, or, equivalently, spacetime.
As in all things, however, there are heretics. One of them is Freeman Dyson, a legend of the field and, for most of his life, distinguished professor at the Institute for Advanced Studies in Princeton. Dyson first showed that if the theory of gravity is General Relativity, it is in principle impossible to detect the quantization of the gravitational field by any real-life apparatus. He took this to mean that there is in fact no quantization, namely that the failures in reconciling General Relativity with Quantum Mechanics are really due to the fact that the gravitational field is a purely classical entity. In the dichotomous world he envisioned, the geometric theory of gravity would peacefully coexist with the quantum realm, and the obstruction in observing individual gravitons would be wholly attributable to their nonexistence.
The central question therefore is the following: do we need to quantize gravity? And if so, is there a way to show experimentally if gravity follows the precepts of quantum mechanics? Our latest research is devoted to this problem.
Dark Lines
In 1814, the Bavarian physicist and optician Joseph von Fraunhofer discovered dark lines in Isaac Newton’s color spectrum. von Fraunhofer didn’t know it at the time, but with that simple observation he basically discovered the quantization of the electromagnetic field. The dark lines, or absorption lines, in the electromagnetic spectrum match the frequencies of the photons that are absorbed by (quantum) matter. For example, if white light shines on a gas of hydrogen, the outgoing electromagnetic wave will contain a dark line at a wavelength of approximately 100 nm, corresponding to the transition of the hydrogen atom as an electron goes from the ground state (the state of minimum energy) to the first excited state by absorbing a photon of that frequency.
Is it possible to do something similar with gravity? In our work, we devise a type of experiment that would be able to discriminate between quantum and classical gravity by looking at the gravitational analogue of electromagnetic absorption lines. If gravitons exist, it should be possible to detect them by shining a gravitational wave on a very dense object, like a neutron star or a black hole, and look for dark lines. Unfortunately, Dyson’s aforementioned argument makes things difficult. Dyson showed that it is impossible to detect a single graviton with high probability in any realistic experiment, and conjectured a censorship effect that precludes the observation of the quantization of the gravitational field in Einstein’s theory. Absorption lines are just another way of probing the quantization of gravity, therefore they are excluded by similar arguments. What this means is that, even if gravity is quantized, it could be impossible to detect it being so just because of the nature of this force. Spacetime looks “unbearably light”, concealing its discrete nature to any type of experiment, no matter how cleverly designed.
Nevertheless, our research shows that gravitational dark lines will appear if General Relativity is modified in the early universe in such a way as to make gravity stronger for the absorption material. Usually, the probability for a particle being absorbed by a generic material is proportional to the area of the target. This is not true for gravity, as the probability of absorbing a graviton in General Relativity is universal, and proportional to the Planck area, the minimum meaningful area that one can consider in any theory of quantum gravity. Any effort in probing smaller distances would collapse that region of space to a microscopic black hole due to the extremely condensed energy required to resolve such a tiny patch of spacetime.
One can modify the mathematical structure of General Relativity to increase the area of the target material, making it significantly bigger than the Planck area, and therefore enhancing the probability of absorption by several orders of magnitude. In this way, gravitons could be absorbed with high probability by primordial atoms existing in the far past, when the universe was much smaller and denser. The dark lines would not show up in ordinary gravitational waves produced by black holes or other astronomical objects, but rather in the primordial gravitational wave spectrum, ripples in spacetime created within a trillionth of a trillionth of a trillionth of a second of the Big Bang.
If discovered, these dark lines would explore physics beyond what is currently known, deep into the pure quantum gravity regime, and they would prove once and for all that gravity is indeed quantized, just like all the other forces.